The Physiology of a Trigger Point
Physicians who haven't taken the time to study trigger points and myofascial pain tend to doubt they exist. Regarding her resistant peers, Janet Travell often spoke with a twinkle in her eye of her need to "rearrange their prejudices". Her enduring aspiration was to scientifically validate the existence of myofascial trigger points and convert the disbelievers. Although far too many physicians still remain unconvinced, Dr. Travell managed to build a very strong scientific basis for the acceptance of this radical new science among those willing to at least tentatively open their minds and consider the evidence. The Validation of Trigger Points
One of Dr. Travell's earliest discoveries occurred in 1957 when she found that trigger points generate tiny electrical currents. This allowed the activity of a trigger point to be quantified by measuring these tiny signals with electromyographic instruments. The precise location of a trigger point could also be determined by this same means. A number of other things were revealed by studying these electrical signals.
Muscle tissue is electrically active only when in a state of contraction. It follows that when electrical activity is confined to a very small area, only a small part of the muscle is contracting. Dr. Travell found that pressing on a trigger point increases its electrical activity. Stretching a muscle did the same thing, which may explain why stretching so often makes pain worse, perhaps by irritating the trigger point (Simons, Travell, and Simons 1999,58-69).
Dr. Travell established that the most convincing practical demonstration of the existence of trigger points was to simply feel for them with the fingers. Active and latent trigger points alike give a distinctively painful response to pressure. If a trigger point is near the surface, sensitive fingers can detect that it's a little warmer than surrounding tissue. This temperature difference, which is due to increased metabolic activity in the trigger point, is measurable (Simons, Travell, and Simons 1999, 29-30).
Being soft tissue, trigger points can't be seen on X-rays. They have been viewed, however, with both electron and light microscopy in fresh human cadavers and in live animal biopsies. In the second edition of volume 1 of the Trigger Point Manual, Travell and Simons include a very elucidating microscopic photograph of a trigger point in a dog's leg muscle (Simons, Travell, and Simons, 1999, 69). Dr. Simons permitted use of the photograph in this book and it appears in the next section (Figure 2.11).
Janet Travell's meticulous scientific investigations have great value for the scientifically minded. For practical purposes, however, all the average person really needs to know about trigger points is that they're extremely sore spots in muscles that send pain to other places. With this uncomplicated idea in mind and trigger point charts in hand, a dedicated individual usually has no trouble finding trigger points and successfully treating them.
For those who feel the need to go deeper into the science of myofascial pain, it can be quite a challenge. The physiology of a trigger point is fascinating, but it's complex. Even so, you can simplify the study of trigger points by looking at them in two separate ways: microscopically and electrochemically. |
The Microscopic View of a Trigger Point
A muscle fiber actually consists of a bundle of several hundred smaller fibers called myofibrils. As seen in Figure 2.8, the muscle fibers themselves make up larger units called fascicles. Numerous fascicles, in turn, are bound together by fascia to form a section of muscle. There are approximately one hundred fibers in a fascicle, and each muscle fiber contains between one thousand and two thousand myofibrils. Interestingly, a myofibril is actually a muscle cell, which because of its unusual length contains more than one nucleus.
The smallest unit of muscle contraction that can be seen at the microscopic level is in a tiny part of the myofibril called a sarcomere (Figures 2.8 and 2.9). Figure 2.9 shows a sarcomere relaxed (A) and contracted (B). Note that the contracted sarcomere is considerably shorter.
Each myofibril is made up of a chain of sarcomeres connected end to end. An element called a Z band separates each sarcomere like a thin wall. Note in Figure 2.9 that the Z bands in the contracted sarcomere have moved closer together. The length of a fully contracted sarcomere can approach half the length of a fully relaxed sarcomere. It's estimated that the average length of an uncontracted human sarcomere is about 1.3 microns, or about 0.00005 inch (Mense and Simons 2001, 252). That's small enough to make a sarcomere seem insignificant, but it's actually where all the action is.
|
The essential parts of each sarcomere are two filament-like protein molecules: a ctin and myosin. Contraction occurs in a sarcomere when the actin and myosin molecules are attracted to one another and come together, somewhat like interlocking your fingers to bring your hands together. This action shortens the sarcomere, which in turn shortens its tiny part of the muscle. The shortening of sarcomeres is the heart of muscle contraction. As you can imagine, millions of sarcomeres have to contract in order to make even the smallest movement. |
Relaxation of a sarcomere happens when the actin and myosin are repelled from one another and pull apart. They never separate completely. Instead they stand ready to re unite at the least urging by an impulse from the nervous system. A muscle can be exhausted by any kind of work or play that requires its sarcomeres to contract and relax too often. A trigger point comes into being when exhaustion causes actin and myosin to become stuck in their interlocked state. This locking up of the sarcomeres occurs about midway along the length of the muscle right where the motor nerve enters. The motor nerve is the means for transmitting the signal telling the muscle to contract (Simons Travelt and Simons 19991 45-57).
Figure 2.10 depicts several muscle fibers within a trigger point in the infraspinatus muscle of the shoulder. The illustration is a representation of a microscope photograph of an actual sarcomere contracture that makes up a trigger point (Figure 2.11). An examination of Figure 2.10 clarifies what is seen in Figure 2.11. |
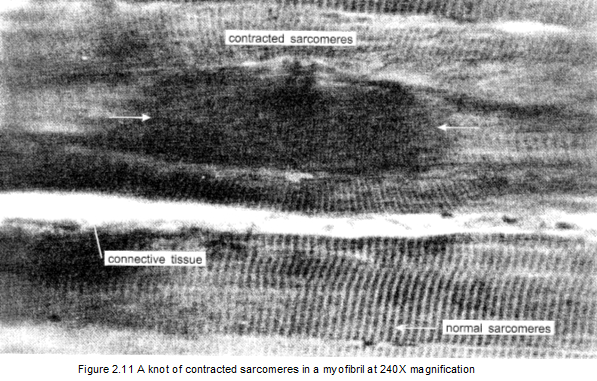 |
Letter A in Figure 2.10 is a muscle myofibril in a normal resting state, neither stretched nor contracted. The tiny vertical Z bands within the fiber mark the ends of individual sarcomeres. The sarcomeres run lengthwise in the fibril.
Letter B is a knot in a myofibril consisting of a mass of sarcomeres in the state of maximum continuous contraction that characterizes a trigger point. The bulbous appearance of the contraction knot indicates how that segment of the myofibril has contracted, with the Z bands of individual sarcomeres now drawn closer together.
Letter C is the part of the myofibril that extends from the contraction knot to the muscle's attachment (to the head of the humerus in this case). Note the greater distance between the vertical Z bands, which shows how the sarcomeres in this part of the myofibril are being stretched by the tension within the contraction knot. These stretched segments are what give tightness and rigidity to the taut band. Therapy should aim at relaxing the knotted sarcomeres, which will allow the stretched sarcomeres along the full length of the fibril to equalize. In this way, the trigger point will release, allowing the taut band to relax.
Normally the action of the sarcomeres, alternately contracting and releasing like tiny pumps, helps the heart circulate blood through the tiny capillaries that supply the sarcomeres' own metabolic needs. When sarcomeres in a trigger point hold their contraction, they squeeze the capillaries shut and blood flow in the area essentially stops. The resulting oxygen starvation and accumulation of the waste products of metabolism causes even more sarcomeres to lock up. When a sufficient number of sarcomeres are disabled, they take on the group identity of a myofascial trigger point, with its characteristic knotlike feel, hypersensitivity, and generation of referred pain (Simons, Travell, and Simons 1999, 57-78).
|
The Electrochemical View of a Trigger Point
Muscle Metabolism The electrochemical processes that occur in the muscles of the body are all part of muscle metabolism, which encompasses the basic functions of contracting and relaxing. Two contrasting processes take place in metabolism: anabolism and catabolism. Anabolism converts nutrients into new body tissue. Catabolism transforms nutrients into energy and heat. The elimination of the waste products of these processes is also part of muscle metabolism, but transforming energy into motion is the primary job. The act of contraction in a muscle and the resultant motion can't occur without muscle metabolism, and metabolism can't occur without an energy source (food).
Metabolism proceeds by transforming the glucose in food into glycogen and fat molecules, which are used for energy storage. When cells need energy, glycogen and fat are converted into the molecule adenosine triphosphate (ATP), which then serves as a carrier of energy, transporting it wherever it's needed.
Adenosine triphosphate is a molecule that consists of three main parts: adenine, ribose, and a chain of three phosphates. Although ribose is a form of sugar, the phosphates are the actual power source. Energy contained in the bonds between the phosphates is released when they are broken, which occurs through hydrolysis, the addition of a water molecule. When the phosphate bonds are broken, energy is produced and one of the three phosphates is removed, leaving a residual molecule, adenosine diphosphate (ADP), with only two phosphates. Interestingly, adenosine diphosphate isn't treated as waste but is processed back into adenosine triphosphate by the addition of phosphate from the diet, thus making very efficient use of the energy source.
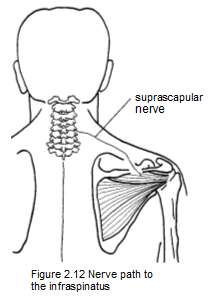
Muscle Contraction
Muscle contraction usually begins as an electrical signal from the brain, although reflexive muscle action needs only an impulse from the spinal cord. In both cases, the signal is taken to the muscle by a motor nerve, which is actually a complex sort of cable containing thousands of individual nerve fibers called axons. In Figure 2.12, the impulse for contraction is carried from the spinal cord to the infraspinatus muscle by the suprascapular nerve.
In a closer view, an axon connects its nerve cell, or neuron, to the individual muscle fibers (Figure 2.13). All of the nerve cells that activate muscle contraction reside in the spinal cord. The sensory nerve cells are just outside the spinal cord in the ganglia, small bulbous e nlargements of the spinal nerves, which exit between the vertebrae. The sensory nerve fibers for the infraspinatus muscle also travel in the
suprascapular nerve. The capillaries that supply blood to the area usually parallel the nerve (Figure 2.14). Note that the axon divides into multiple parts and ends just short of the muscle fiber in what is called the motor endplate zone. This is about halfway along the muscle fiber's length, midway between its attachments.
The tiny gap between the motor end plate and the muscle fiber is termed the synaptic cleft. This is where the electrical signal of the nerve fiber is converted to the chemical messenger acetylcholine, which carries the electrical signal to the cell membrane of the muscle fiber. To stimulate contraction, this signal is immediately disseminated throughout the entire muscle fiber.
|
Figure 2.15 shows an individual neuromuscular junction before the release of acetylcholine, when the sarcomeres are at their full length and relaxed. The mitochondria produce the acetylcholine, which is then transported by the acetylcholine vesicles. Figure 2.16 shows how the flow of acetylcholine affects the sarcomeres, causing them to contract and shorten. Acetylcholine is the critical substance in producing contraction of the sarcomeres in the muscles. When the release of acetylcholine gets out of controt muscle contraction also gets out of control. Muscle overload, muscle tension, and sustained rapid movement can all cause excessive release of acetylcholine. This in turn causes sarcomeres to become locked up and unresponsive, beginning what Travell and Simons call an "energy crisis" (Simons, Travell, and Simons 1999, 69-75).
|
|
|
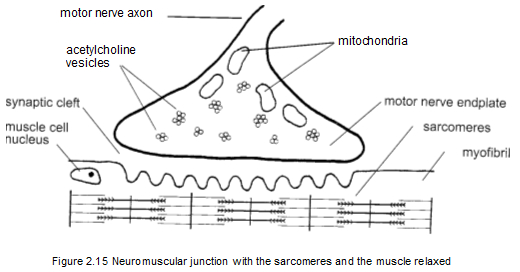 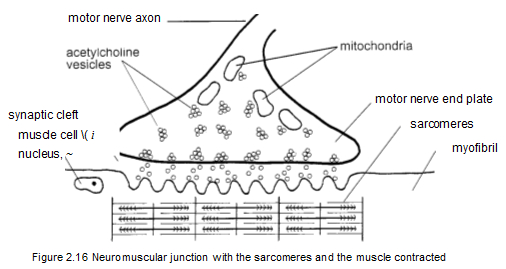 |
|
The energie crisis Travell and Simons's energy crisis constitutes a vicious cycle of occurrences at the neuromuscular junction that's believed to be the basis for formation of myofascial trigger points (Figure 2.17). The problem begins with muscle overuse or sustained contraction, 'which constricts the capillaries that supply the muscle's metabolic needs. Circulation in a muscle fails during a sustained contraction that is more than 30 to 50 percent of maximum effort (Simons, Travell, and Simons 1999, 71). Failed circulation then causes decreased delivery of ATP, the energy molecule. Since energy is needed to detach the myosin filaments from the actin filaments, the absence of ATP leaves the sarcomeres in a shortened and contracted state (Simons, Travell, and Simons 1999, 69-75).
The key process in the energy crisis occurs when reduced ATP stops the reuptake of calcium. This is important because the sarcomeres can't relax and lengthen as long as calcium is present. Calcium ions are the immediate mediators of contraction, being the substance that directly stimulates the actin and myosin elements to link up and shorten. Calcium also acts in the synaptic cleft of the nerve terminal to cause the release of acetylcholine. Because of this, reducing the calcium reuptake leaves an excessive concentration of calcium in the synaptic cleft, promoting an overabundance of acetylcholine, which in turn contributes to maintaining the contraction. This condition at the neuromuscular junction, known as a dysfunctional endplate, is the piece that completes the vicious cycle that creates and perpetuates trigger points (Simons, Travell, and Simons 1999, 70-71).
Breaking the Cycle
A trigger point can be deactivated by almost any technique that causes the myosin filaments to release the actin filaments, thereby allowing the sarcomeres to lengthen. However, the muscle can't be forced to relax, nor can it be forced to lengthen without the risk of stimulating further release of acetylcholine. The safest and most effective way to break the vicious cycle that maintains the trigger point is to increase blood circulation, which very quickly increases the supply of oxygen and energy to the muscle tissues.
With the restoration of the energy supply, the reuptake of calcium will begin again, allowing the myosin and actin molecules to part and the sarcomeres to lengthen. Massage of the trigger point is the most direct and risk-free means for establishing renewed circulation through the capillaries in the affected area (Simons, TravelL and Simons 1999, 141-142).
|
|
|